Section 1: Introduction to Synthetic Biology
Definition and Overview
What is Synthetic Biology?
Synthetic biology is an interdisciplinary field that combines biology, engineering, and computer science to design and construct new biological parts, devices, and systems. It also involves the redesign of existing natural biological systems for useful purposes. The field aims to apply engineering principles to biology, enabling the creation of standardized biological components that can be used to build complex systems. This approach allows scientists to engineer organisms with novel functions, leading to innovations in medicine, agriculture, industry, and environmental management.
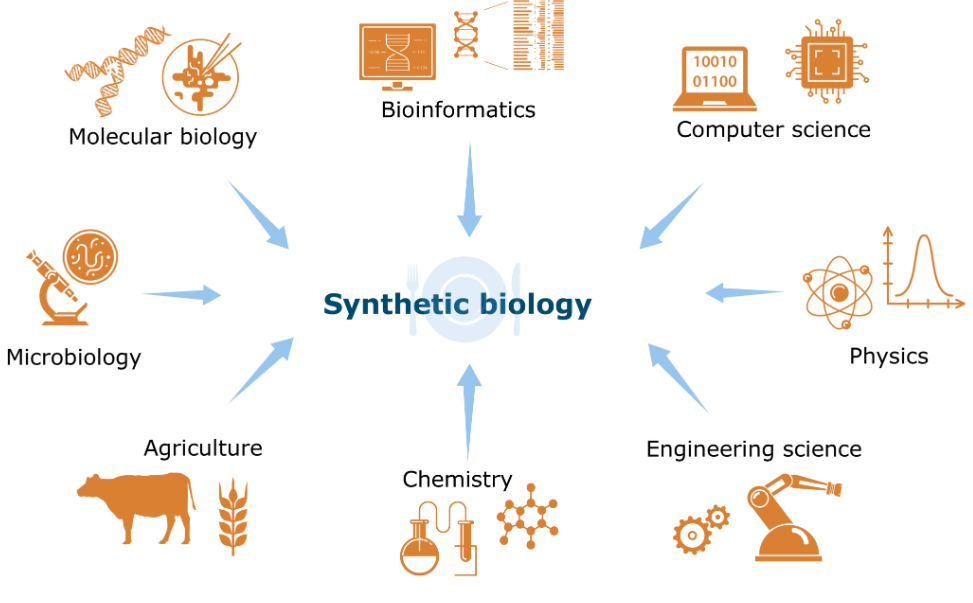
Historical Development and Milestones
The origins of synthetic biology can be traced back to the early 2000s, but its conceptual roots extend to the dawn of genetic engineering in the 1970s. Key milestones include the development of recombinant DNA technology and the Human Genome Project. In 2003, the J. Craig Venter Institute synthesized a minimal bacterial genome, a landmark achievement demonstrating the potential to design life from scratch. Over the past two decades, advancements in DNA synthesis, gene editing, and computational biology have accelerated progress, transforming synthetic biology into a dynamic and rapidly evolving field.
Key Concepts and Terminologies
Synthetic biology involves several core concepts and terminologies, such as modularity, standardization, and abstraction. Modularity refers to the design of biological systems using standardized, interchangeable parts known as “BioBricks.” These components can be assembled into larger systems with predictable behaviors. Abstraction layers, including parts, devices, and systems, simplify the design process, allowing researchers to focus on specific levels of complexity. The development of these principles has been critical to the growth of synthetic biology, enabling the creation of increasingly sophisticated biological systems.
Foundational Principles
Principles of Genetic Engineering
Genetic engineering is a fundamental aspect of synthetic biology, involving the direct manipulation of an organism’s DNA to alter its characteristics. Techniques such as gene cloning, DNA sequencing, and polymerase chain reaction (PCR) form the backbone of genetic engineering. In synthetic biology, these techniques are used to construct new genetic sequences or modify existing ones to introduce desired traits. The ability to precisely edit genomes is central to synthetic biology, enabling the creation of custom-designed organisms with specific functions.
Biological Systems and Design
Synthetic biology applies engineering principles to the design and construction of biological systems. This involves defining biological parts with specific functions, such as promoters, ribosome binding sites, and coding sequences. These parts can be combined to create devices like genetic circuits and metabolic pathways. The design process often employs computer-aided design (CAD) tools and simulation software to model and predict system behavior. By applying these principles, researchers can engineer organisms that perform complex tasks, such as producing biofuels or detecting environmental pollutants.
Modularity, Abstraction, and Standardization
The concepts of modularity, abstraction, and standardization are central to synthetic biology. Modularity allows biological systems to be constructed from standardized parts, enabling researchers to build complex systems with predictable behaviors. Abstraction layers simplify the design process by providing different levels of detail, from individual parts to entire systems. Standardization involves creating uniform protocols and specifications for biological parts, ensuring compatibility and interoperability. These principles have facilitated the growth of synthetic biology, enabling researchers to rapidly design, build, and test new biological systems.
Section 2: Core Techniques in Synthetic Biology
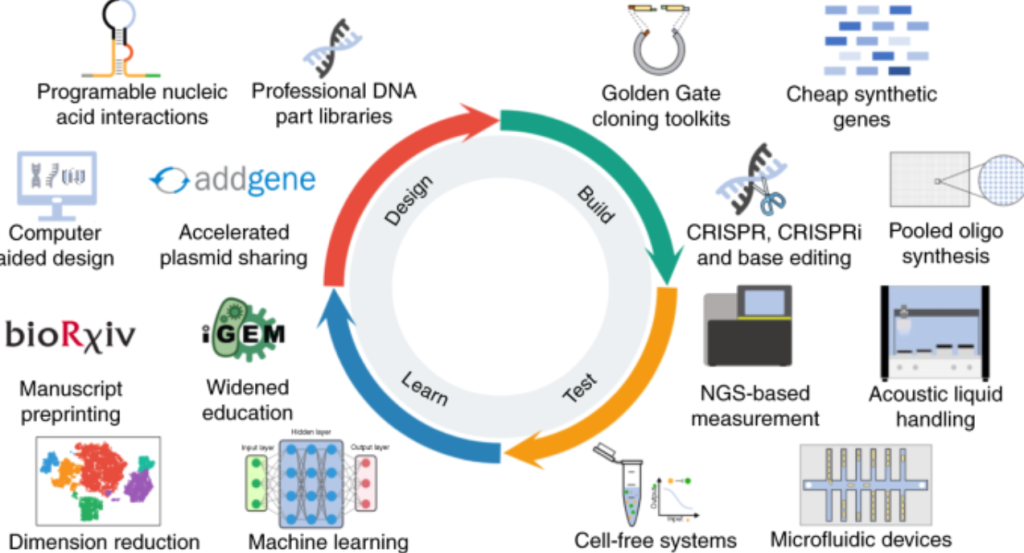
Genetic Engineering Tools
CRISPR-Cas9 and Gene Editing
CRISPR-Cas9 is a revolutionary gene-editing technology that enables precise modifications to DNA sequences. This system uses a guide RNA to target specific DNA sequences, while the Cas9 enzyme introduces double-strand breaks at the target site. The cell’s repair mechanisms then modify the DNA, either by disrupting the gene or inserting new sequences. CRISPR-Cas9 has transformed synthetic biology by providing a powerful, versatile tool for genome editing, with applications ranging from basic research to developing genetically modified organisms with enhanced traits.
Recombinant DNA Technology
Recombinant DNA technology is a cornerstone of synthetic biology, allowing scientists to combine DNA from different sources to create new genetic constructs. This process involves isolating and cutting DNA fragments using restriction enzymes, ligating them into vectors, and introducing them into host organisms. The recombinant DNA is then expressed, producing new proteins or traits. This technique has enabled the development of genetically modified organisms, such as bacteria that produce insulin or crops with increased resistance to pests.
Gibson Assembly and Golden Gate Assembly
Gibson Assembly and Golden Gate Assembly are advanced techniques for assembling multiple DNA fragments into a single construct. Gibson Assembly uses exonucleases, DNA polymerase, and ligase to join overlapping DNA fragments, enabling the construction of large and complex genetic sequences. Golden Gate Assembly employs type IIs restriction enzymes to generate compatible overhangs, allowing the seamless assembly of multiple fragments in a defined order. These methods streamline the construction of genetic constructs, accelerating the design and testing of synthetic biology applications.
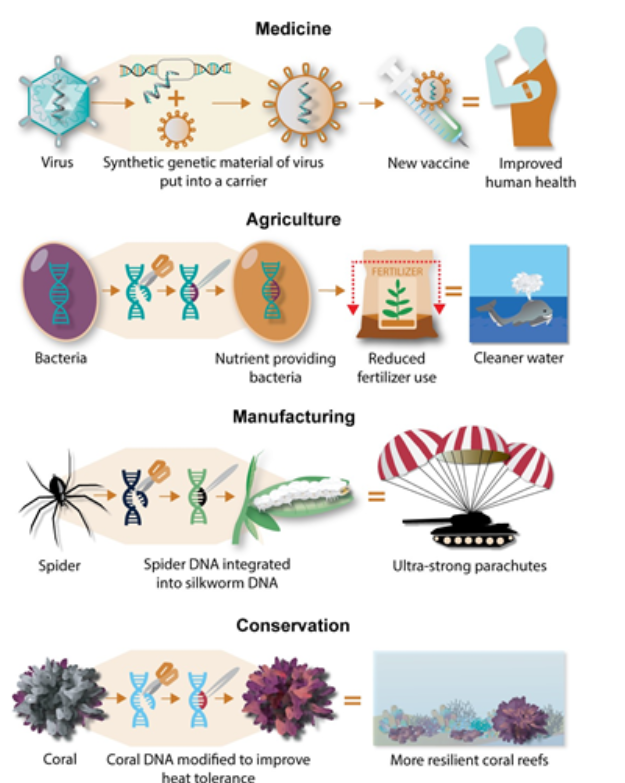
Synthetic Gene Networks
Gene Circuits and Logic Gates
Synthetic gene networks are designed to perform specific functions within living cells, similar to electronic circuits. These networks use genetic components such as promoters, ribosome binding sites, and coding sequences to create circuits that process biological signals. Logic gates, such as AND, OR, and NOT gates, are fundamental building blocks of gene circuits, enabling the creation of complex regulatory networks. By engineering gene circuits, researchers can program cells to perform tasks like sensing environmental changes or producing therapeutic compounds in response to specific stimuli.
Promoter Engineering
Promoter engineering involves modifying promoter sequences to control gene expression levels. Promoters are DNA sequences that initiate transcription, and their strength and specificity can be altered to fine-tune gene expression. Techniques such as random mutagenesis and directed evolution are used to create promoter libraries with varying activities. By selecting promoters with desired characteristics, synthetic biologists can precisely regulate gene expression in engineered organisms, optimizing the performance of synthetic gene networks.
Regulatory Elements and Switches
Regulatory elements and switches are critical components of synthetic gene networks, enabling dynamic control of gene expression. These elements include enhancers, repressors, and riboswitches, which modulate transcription or translation in response to specific signals. Synthetic biologists design regulatory switches to control gene expression in a context-dependent manner, allowing engineered organisms to adapt to changing environments. By incorporating these elements into synthetic gene networks, researchers can create sophisticated biological systems with precise control over cellular behavior.
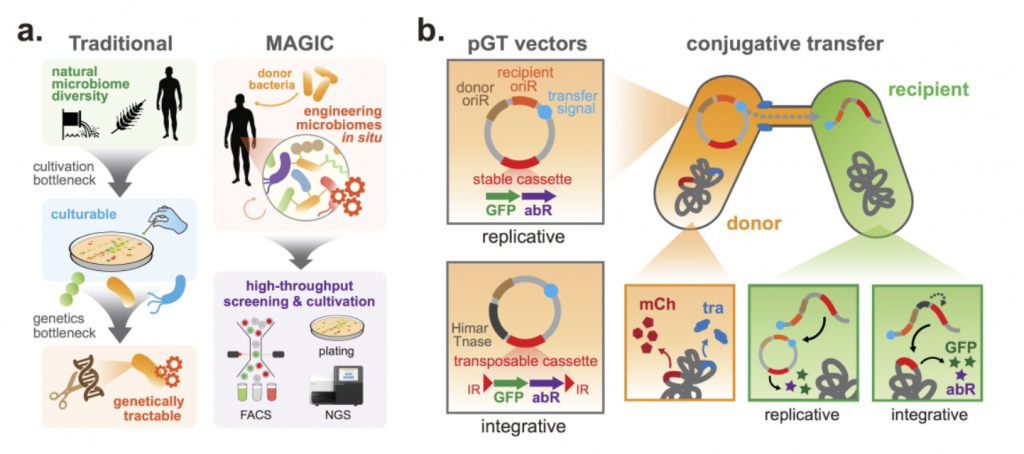
Genome Synthesis and Editing
De Novo Genome Synthesis
De novo genome synthesis involves constructing entire genomes from scratch, enabling the creation of custom-designed organisms. This process begins with the chemical synthesis of DNA oligonucleotides, which are assembled into larger fragments and ultimately into complete genomes. De novo synthesis allows researchers to design genomes with novel traits or minimal complexity, providing insights into fundamental biological processes. This approach has been used to create synthetic bacteria with streamlined genomes and engineered yeast strains with enhanced metabolic capabilities.
Genome Editing Techniques
Genome editing techniques enable precise modifications to existing genomes, allowing researchers to introduce, delete, or alter specific genes. In addition to CRISPR-Cas9, other techniques such as zinc-finger nucleases (ZFNs) and transcription activator-like effector nucleases (TALENs) are used for targeted genome editing. These tools provide synthetic biologists with the ability to engineer organisms with enhanced traits, such as disease resistance or increased productivity, and to study the functions of specific genes in complex biological systems.
Applications in Microorganisms, Plants, and Animals
Synthetic biology techniques are applied across diverse organisms, including microorganisms, plants, and animals. In microorganisms, synthetic biology is used to engineer bacteria and yeast for applications in biotechnology, such as biofuel production and pharmaceuticals. In plants, synthetic biology enables the development of crops with improved traits, such as drought resistance or enhanced nutritional content. In animals, synthetic biology holds promise for applications in agriculture, conservation, and medicine, including the development of animal models for disease research and the production of therapeutic proteins.
Section 3: Applications of Synthetic Biology
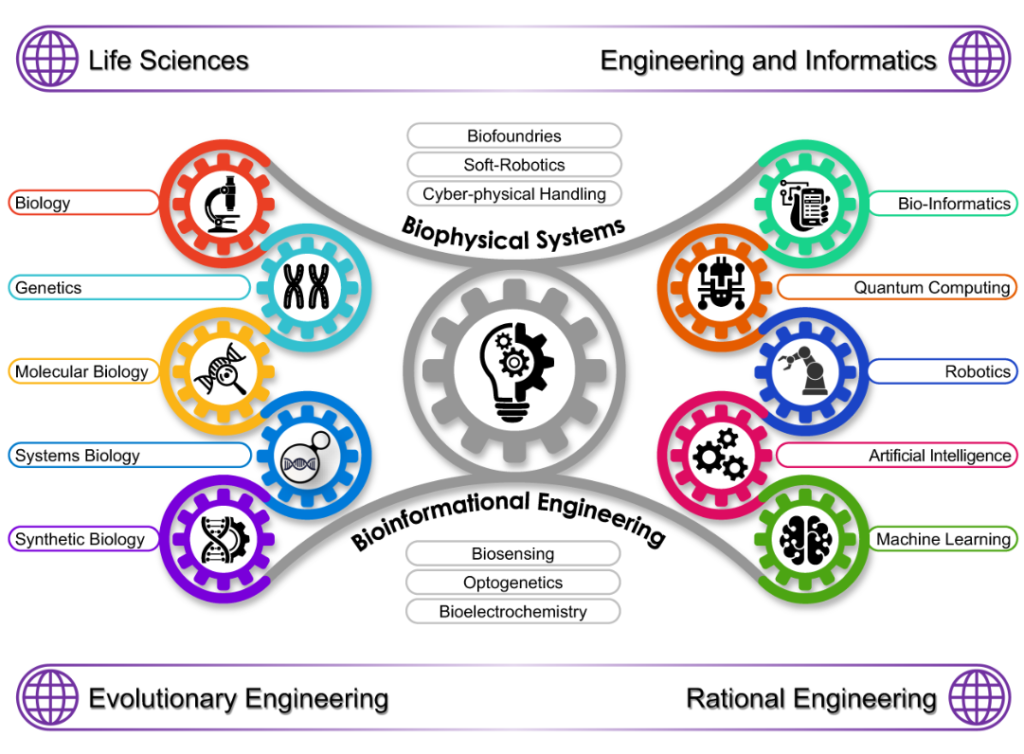
Healthcare and Medicine
Synthetic Biology in Drug Development
Synthetic biology is transforming drug development by enabling the design and production of novel therapeutics. By engineering microorganisms to produce complex molecules, synthetic biology accelerates the discovery and synthesis of new drugs, such as antibiotics and anticancer agents. Additionally, synthetic biology techniques are used to optimize drug delivery systems and develop targeted therapies, improving treatment efficacy and reducing side effects.
Gene Therapy and Personalized Medicine
Gene therapy involves using synthetic biology tools to correct genetic defects and treat diseases at the molecular level. By delivering functional genes or editing defective ones, synthetic biology offers the potential to cure genetic disorders and provide personalized treatments. Advances in gene editing technologies, such as CRISPR-Cas9, are driving the development of personalized medicine, where treatments are tailored to an individual’s genetic makeup, improving outcomes and minimizing adverse effects.
Synthetic Biology for Vaccine Development
Synthetic biology is revolutionizing vaccine development by enabling the rapid design and production of vaccines against emerging pathogens. By engineering microorganisms to produce vaccine antigens or using synthetic gene circuits to enhance immune responses, synthetic biology accelerates vaccine development and production. This approach has been instrumental in the response to infectious diseases, such as COVID-19, where synthetic biology-enabled platforms have facilitated the rapid development of effective vaccines.
Agriculture and Food
Genetically Modified Crops
Synthetic biology is advancing agriculture by enabling the development of genetically modified crops with enhanced traits, such as increased yield, pest resistance, and improved nutritional content. By engineering plant genomes, synthetic biologists can introduce beneficial traits, such as drought tolerance or resistance to specific pathogens, improving crop productivity and resilience. Genetically modified crops have the potential to address global food security challenges by increasing agricultural efficiency and sustainability.
Synthetic Biology in Food Production
Synthetic biology is driving innovation in food production by enabling the development of alternative proteins and sustainable food sources. By engineering microorganisms to produce proteins, fats, and other food components, synthetic biology offers a sustainable approach to food production that reduces the environmental impact of traditional agriculture. This technology is being used to develop plant-based meats, dairy alternatives, and other novel food products, providing consumers with sustainable and nutritious options.
Biofortification and Nutrient Enhancement
Biofortification involves using synthetic biology to enhance the nutritional content of crops, addressing micronutrient deficiencies in populations worldwide. By engineering plants to produce higher levels of vitamins, minerals, and other essential nutrients, synthetic biology offers a sustainable approach to improving public health. Biofortified crops, such as rice enriched with vitamin A or iron-fortified maize, have the potential to alleviate malnutrition and improve health outcomes in vulnerable communities.
Industrial Biotechnology
Biofuels and Bioplastics
Synthetic biology is enabling the production of biofuels and bioplastics by engineering microorganisms to convert renewable resources into valuable products. By optimizing metabolic pathways and introducing novel enzymes, synthetic biologists can enhance the efficiency and yield of biofuel production, reducing reliance on fossil fuels and decreasing greenhouse gas emissions. Similarly, synthetic biology techniques are used to produce biodegradable plastics, offering sustainable alternatives to petroleum-based materials.
Biomanufacturing and Biosynthesis
Biomanufacturing involves using synthetic biology to produce high-value compounds, such as pharmaceuticals, chemicals, and materials, through microbial fermentation. By engineering microorganisms to produce complex molecules, synthetic biology offers a cost-effective and environmentally friendly approach to manufacturing. This technology is being used to produce a wide range of products, from specialty chemicals and enzymes to fragrances and flavors, enabling sustainable and scalable production processes.
Metabolic Engineering
Metabolic engineering involves optimizing cellular metabolism to enhance the production of specific compounds, such as biofuels, pharmaceuticals, or industrial chemicals. By modifying metabolic pathways and introducing novel enzymes, synthetic biologists can improve the efficiency and yield of bioprocesses. This approach enables the sustainable production of valuable products from renewable resources, reducing the environmental impact of traditional manufacturing processes and contributing to a circular economy.
Environmental Applications
Bioremediation and Pollution Control
Synthetic biology offers innovative solutions for environmental remediation by engineering microorganisms to degrade pollutants and restore ecosystems. By introducing genes encoding enzymes that break down toxic compounds, synthetic biologists can develop microorganisms capable of detoxifying contaminated environments, such as oil spills or industrial waste sites. This approach offers a sustainable and effective method for pollution control, contributing to environmental conservation and protection.
Biosensors for Environmental Monitoring
Biosensors are analytical devices that use biological components to detect specific analytes or environmental conditions. Synthetic biology enables the development of biosensors with enhanced sensitivity and specificity by engineering microorganisms or proteins to respond to target compounds. These biosensors can be used for environmental monitoring, detecting pollutants, pathogens, or changes in environmental conditions, providing valuable data for decision-making and resource management.
Synthetic Biology for Conservation
Synthetic biology holds potential for conservation efforts by enabling the preservation of endangered species and ecosystems. By engineering organisms to enhance resilience or resistance to environmental stressors, synthetic biology can support biodiversity conservation and habitat restoration. Additionally, synthetic biology techniques are being explored for de-extinction, where extinct species are revived through genetic reconstruction, offering new tools for conservation science.
Section 4: Tools and Platforms for Synthetic Biology
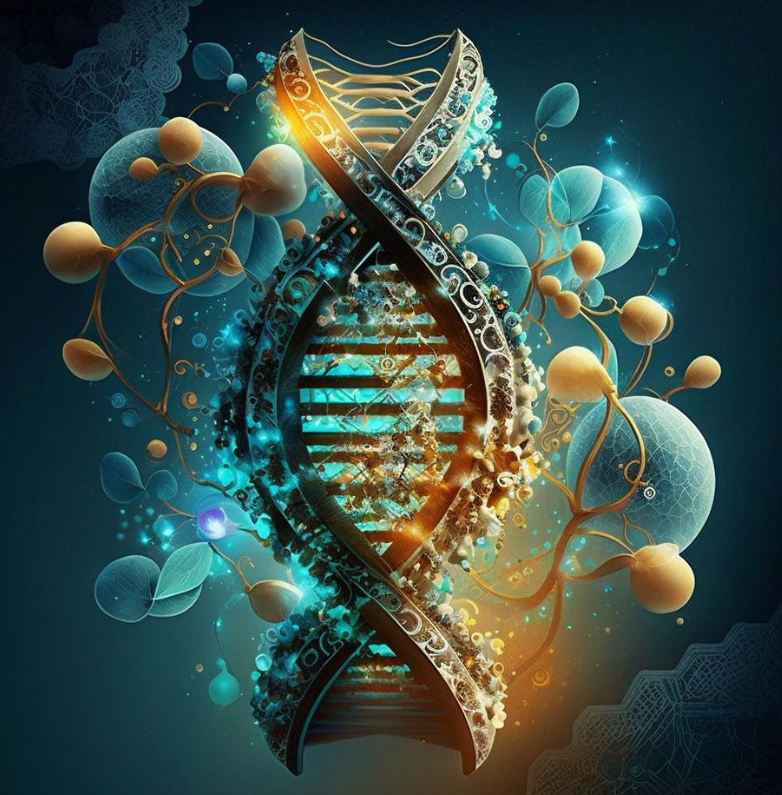
Software and Computational Tools
Bioinformatics and Data Analysis
Bioinformatics is a key component of synthetic biology, providing tools and techniques for analyzing biological data and designing synthetic systems. Bioinformatics platforms enable researchers to analyze genomic sequences, predict protein structures, and model metabolic pathways, facilitating the design and optimization of synthetic biology applications. By integrating computational tools with experimental workflows, synthetic biologists can accelerate the design and testing of engineered organisms.
Design and Simulation Software
Design and simulation software play a crucial role in synthetic biology, enabling researchers to model and predict the behavior of synthetic systems. Tools such as SBOL (Synthetic Biology Open Language), CAD (Computer-Aided Design), and simulation platforms like COPASI and CellDesigner allow researchers to design genetic constructs, simulate gene circuits, and optimize metabolic pathways. These tools enhance the efficiency and accuracy of synthetic biology research, reducing the need for trial-and-error experimentation.
Synthetic Biology Databases
Synthetic biology databases provide access to a wealth of information on genetic parts, devices, and systems, facilitating the design and construction of synthetic organisms. Resources such as the Registry of Standard Biological Parts, iGEM’s BioBrick database, and Addgene’s plasmid repository offer standardized components for synthetic biology applications. These databases support collaboration and knowledge sharing within the synthetic biology community, accelerating innovation and discovery.
Laboratory Techniques and Equipment
DNA Sequencing and Synthesis
DNA sequencing and synthesis are fundamental techniques in synthetic biology, enabling the characterization and construction of genetic sequences. Next-generation sequencing (NGS) technologies provide high-throughput, cost-effective methods for sequencing entire genomes, while DNA synthesis services offer rapid and accurate production of custom-designed sequences. These techniques are essential for engineering organisms, validating genetic constructs, and optimizing synthetic biology applications.
High-Throughput Screening
High-throughput screening (HTS) is a powerful technique for evaluating the performance of synthetic biology constructs, allowing researchers to test large numbers of genetic variants simultaneously. HTS platforms use automated equipment and robotics to conduct experiments on a massive scale, generating data on gene expression, protein function, and metabolic activity. This approach accelerates the discovery and optimization of synthetic biology applications, enabling researchers to identify high-performing constructs and streamline development.
Automation and Robotics in Synthetic Biology
Automation and robotics are transforming synthetic biology by enabling the rapid and efficient execution of complex experimental workflows. Automated liquid handling systems, robotic workstations, and laboratory information management systems (LIMS) streamline the design, assembly, and testing of synthetic constructs, reducing manual labor and improving reproducibility. By integrating automation with synthetic biology research, scientists can accelerate discovery, increase throughput, and enhance data quality.
Section 5: Ethical, Legal, and Social Implications
Ethical Considerations
Biosecurity and Biosafety
Biosecurity and biosafety are critical considerations in synthetic biology, ensuring that engineered organisms do not pose risks to human health or the environment. Researchers must implement robust containment measures and risk assessments to prevent accidental release or misuse of synthetic organisms. Regulatory frameworks and international guidelines, such as the Cartagena Protocol on Biosafety, provide oversight and guidance for synthetic biology research, balancing innovation with safety.
Ethical Debates in Synthetic Biology
Synthetic biology raises important ethical questions related to the modification and creation of life forms. Debates focus on the moral implications of engineering organisms, the potential impact on biodiversity, and the risks of unintended consequences. Engaging stakeholders, including scientists, ethicists, policymakers, and the public, in open dialogue is essential for addressing these ethical concerns and ensuring responsible innovation in synthetic biology.
Public Perception and Acceptance
Public perception and acceptance of synthetic biology play a crucial role in its development and application. Communicating the benefits, risks, and ethical considerations of synthetic biology to diverse audiences is essential for building trust and fostering informed decision-making. Public engagement initiatives, such as citizen science projects and science communication efforts, help demystify synthetic biology and promote understanding and acceptance of this transformative technology.
Regulatory Frameworks
International Guidelines and Standards
International guidelines and standards provide a framework for the responsible conduct of synthetic biology research and application. Organizations such as the International Genetically Engineered Machine (iGEM) Foundation, the Organization for Economic Cooperation and Development (OECD), and the World Health Organization (WHO) develop guidelines to ensure safety, ethical conduct, and international collaboration in synthetic biology. Adhering to these guidelines is essential for maintaining public trust and enabling the safe and sustainable development of synthetic biology technologies.
Intellectual Property in Synthetic Biology
Intellectual property (IP) plays a significant role in synthetic biology, influencing innovation, commercialization, and access to technology. Patents, copyrights, and trade secrets protect synthetic biology inventions, but IP issues can create challenges related to access, sharing, and collaboration. Balancing IP protection with open innovation and collaboration is crucial for fostering the growth and impact of synthetic biology, enabling researchers to build on each other’s work and accelerate discovery.
Regulatory Challenges and Opportunities
Regulatory challenges in synthetic biology include ensuring safety, managing risks, and addressing ethical concerns. Developing flexible and adaptive regulatory frameworks that accommodate rapid technological advancements while maintaining safety and ethical standards is essential. These frameworks must balance innovation with public trust, enabling the responsible development and deployment of synthetic biology technologies. Opportunities exist for harmonizing international regulations, fostering collaboration, and facilitating the global growth of synthetic biology.
Section 6: Future Trends and Challenges
Emerging Technologies
Synthetic Genomics and Minimal Genomes
Synthetic genomics involves designing and constructing synthetic genomes to create organisms with novel functions or minimal complexity. Advances in DNA synthesis and genome editing enable researchers to create minimal genomes, stripping organisms down to their essential genetic components. These minimal genomes provide insights into the fundamental requirements for life and serve as platforms for engineering organisms with customized traits, offering exciting opportunities for biotechnology and synthetic biology.
Synthetic Biology and Artificial Intelligence
The integration of synthetic biology and artificial intelligence (AI) is driving innovation and accelerating discovery. AI algorithms are used to analyze complex biological data, predict the behavior of synthetic systems, and optimize genetic designs. Machine learning techniques enable researchers to identify patterns and relationships in large datasets, facilitating the development of more efficient and effective synthetic biology applications. The synergy between synthetic biology and AI holds potential for transformative advancements in fields such as healthcare, agriculture, and environmental management.
Nanotechnology in Synthetic Biology
Nanotechnology is increasingly being integrated with synthetic biology to develop novel materials, devices, and systems. By engineering nanoscale structures with specific properties, researchers can create biomimetic materials, drug delivery systems, and biosensors with enhanced performance. The combination of synthetic biology and nanotechnology offers new possibilities for precision medicine, environmental monitoring, and energy production, driving the development of innovative solutions to global challenges.
Challenges and Limitations
Technical Challenges and Limitations
Synthetic biology faces several technical challenges, including the complexity of biological systems, variability in genetic expression, and limitations in predictive modeling. Understanding and controlling these factors are critical for the successful design and implementation of synthetic biology applications. Advances in computational tools, high-throughput screening, and systems biology are helping researchers overcome these challenges, enabling the development of more reliable and robust synthetic systems.
Scaling and Commercialization
Scaling synthetic biology innovations from the laboratory to commercial production presents challenges related to cost, efficiency, and regulatory compliance. Developing scalable bioprocesses, optimizing resource utilization, and ensuring product quality are essential for successful commercialization. Collaborations between academia, industry, and regulatory agencies can facilitate the translation of synthetic biology innovations into market-ready products, driving economic growth and societal benefit.
Addressing Ethical and Societal Concerns
Addressing ethical and societal concerns is crucial for the responsible development and application of synthetic biology. Engaging with stakeholders, including scientists, policymakers, ethicists, and the public, in open dialogue is essential for building trust and ensuring that synthetic biology technologies align with societal values and priorities. By fostering transparency, inclusivity, and collaboration, researchers can address ethical concerns and maximize the positive impact of synthetic biology on society.
Section 7: Case Studies and Success Stories
Pioneering Projects and Innovations
Successful Applications in Medicine
Synthetic biology has led to groundbreaking innovations in medicine, including the development of synthetic insulin, engineered immune cells for cancer therapy, and synthetic vaccines for infectious diseases. These successes demonstrate the potential of synthetic biology to transform healthcare, offering new treatment options and improving patient outcomes.
Breakthroughs in Agriculture and Industry
Synthetic biology has driven significant advancements in agriculture and industry, such as the development of drought-resistant crops, microbial production of biofuels, and biomanufacturing of sustainable materials. These innovations contribute to global sustainability by reducing environmental impact and increasing resource efficiency.
Synthetic Biology Startups and Initiatives
A growing number of startups and initiatives are leveraging synthetic biology to develop innovative solutions across diverse sectors. Companies such as Ginkgo Bioworks, Zymergen, and Twist Bioscience are pioneering new approaches to biomanufacturing, materials production, and healthcare, demonstrating the commercial potential of synthetic biology and driving economic growth.
Lessons Learned and Best Practices
Key Takeaways from Case Studies
Case studies of successful synthetic biology applications offer valuable insights into the challenges and opportunities of the field. Key takeaways include the importance of interdisciplinary collaboration, the need for robust regulatory frameworks, and the value of public engagement and education in building trust and acceptance.
Insights into Successful Implementation
Successful implementation of synthetic biology innovations requires a combination of technical expertise, strategic partnerships, and effective communication. By leveraging the strengths of diverse stakeholders, including researchers, industry leaders, and policymakers, synthetic biology initiatives can overcome obstacles and achieve impactful outcomes.
Strategies for Overcoming Challenges
Overcoming challenges in synthetic biology requires a proactive approach to risk management, ethical considerations, and regulatory compliance. Strategies for success include fostering a culture of innovation, investing in research and development, and engaging with stakeholders to address societal concerns and ensure responsible use of synthetic biology technologies.
Section 8: Hands-On Experiments and Projects
DIY Synthetic Biology Projects
Simple Experiments for Beginners
DIY synthetic biology projects offer accessible and educational opportunities for beginners to explore the principles of synthetic biology. Simple experiments, such as bacterial transformation with fluorescent proteins or gene expression assays, introduce key techniques and concepts, providing hands-on experience and fostering a deeper understanding of synthetic biology.
Building Synthetic Gene Circuits
Building synthetic gene circuits allows enthusiasts and students to explore the design and function of genetic networks. By assembling genetic components, such as promoters and repressors, into functional circuits, learners can investigate gene regulation, logic gates, and cellular responses, gaining practical insights into synthetic biology applications.
Exploring Microbial Systems
Microbial systems offer a versatile platform for synthetic biology experimentation, enabling the study of metabolic engineering, bioremediation, and biosensor development. DIY projects involving microbial systems provide opportunities to explore the engineering of microorganisms for specific functions, such as producing biofuels, degrading pollutants, or detecting environmental changes.
Educational Resources and Workshops
Online Courses and Tutorials
Online courses and tutorials offer structured learning opportunities for individuals interested in synthetic biology. Platforms such as Coursera, edX, and MIT OpenCourseWare provide comprehensive courses on synthetic biology, covering topics such as genetic engineering, metabolic pathways, and bioinformatics, enabling learners to acquire knowledge and skills at their own pace.
Synthetic Biology Competitions (iGEM)
The International Genetically Engineered Machine (iGEM) competition is a prestigious synthetic biology event that brings together students from around the world to design and implement innovative projects. iGEM provides a platform for interdisciplinary collaboration, creativity, and learning, fostering the next generation of synthetic biologists and driving advancements in the field.
Community Laboratories and Biohacking
Community laboratories and biohacking spaces offer resources and support for individuals interested in exploring synthetic biology outside traditional academic settings. These spaces provide access to equipment, materials, and expertise, enabling enthusiasts to conduct experiments, collaborate on projects, and contribute to the growth and democratization of synthetic biology.
Section 9: Resources and Further Reading
Books and Journals
Recommended Reading for Beginners
Books such as “Synthetic Biology: A Primer” by Paul S. Freemont and Richard I. Kitney, and “Adventures in Synthetic Biology” by Drew Endy and Isadora Deese offer accessible introductions to synthetic biology concepts and applications, providing valuable insights for beginners and enthusiasts.
Key Journals and Publications in Synthetic Biology
Journals such as “Nature Communications,” “ACS Synthetic Biology,” and “Journal of Biological Engineering” publish cutting-edge research and reviews in synthetic biology, offering insights into the latest advancements, methodologies, and applications in the field.
Online Courses and Platforms
MOOCs and Online Learning Resources
Massive Open Online Courses (MOOCs) and online learning platforms offer comprehensive courses on synthetic biology, providing learners with access to expert-led instruction, interactive content, and practical exercises. Platforms such as Coursera, edX, and Khan Academy offer courses covering a wide range of topics, from basic genetics to advanced synthetic biology techniques.
Webinars and Conferences
Webinars and conferences provide opportunities for knowledge sharing, networking, and collaboration within the synthetic biology community. Events such as the Synthetic Biology Congress and the Global Synthetic Biology Summit bring together experts, researchers, and industry leaders to discuss emerging trends, challenges, and opportunities in the field.
Research Papers and Reviews
Foundational Papers in Synthetic Biology
Foundational papers such as “Construction of a genetic toggle switch in Escherichia coli” by Gardner et al., and “Synthetic Biology: Applications Come of Age” by Khalil and Collins offer insights into the early developments and foundational concepts of synthetic biology, providing a basis for understanding the evolution and impact of the field.